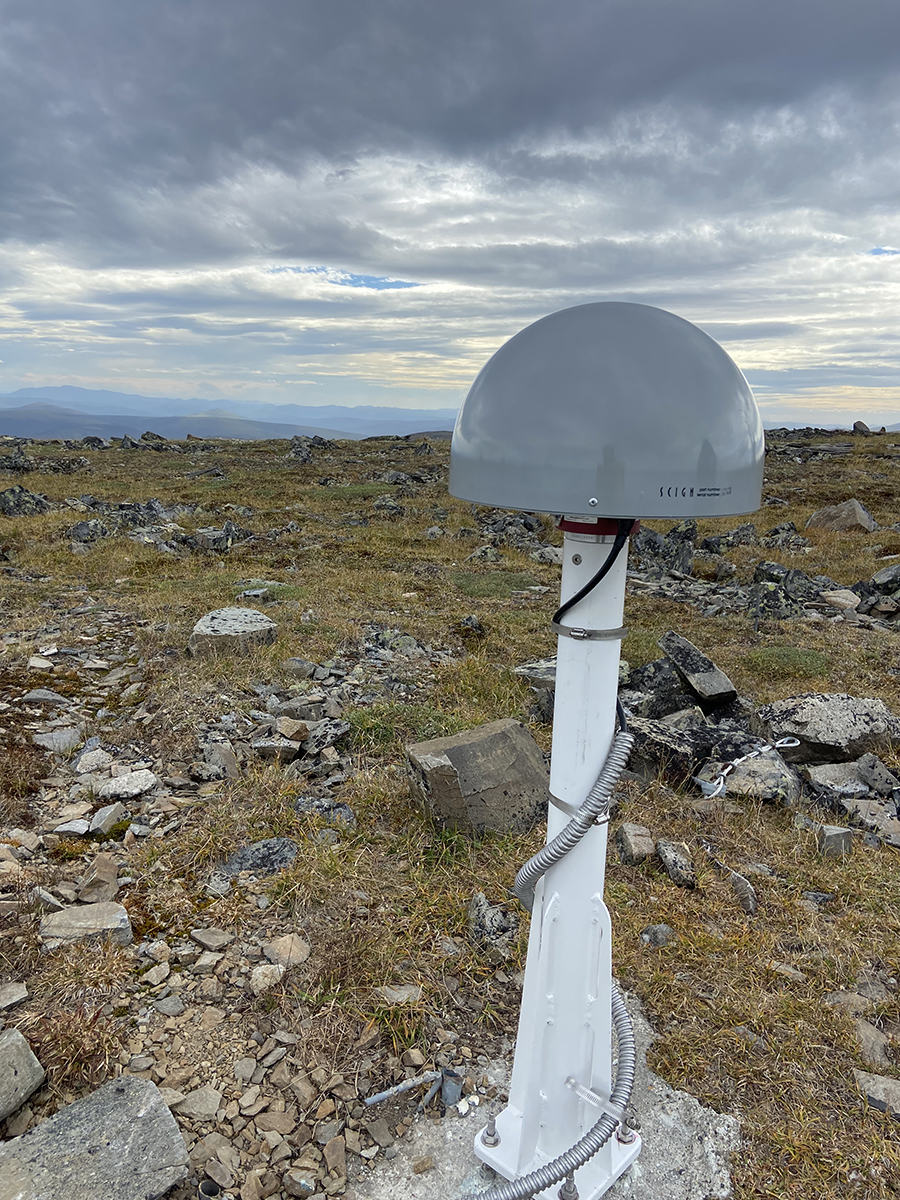
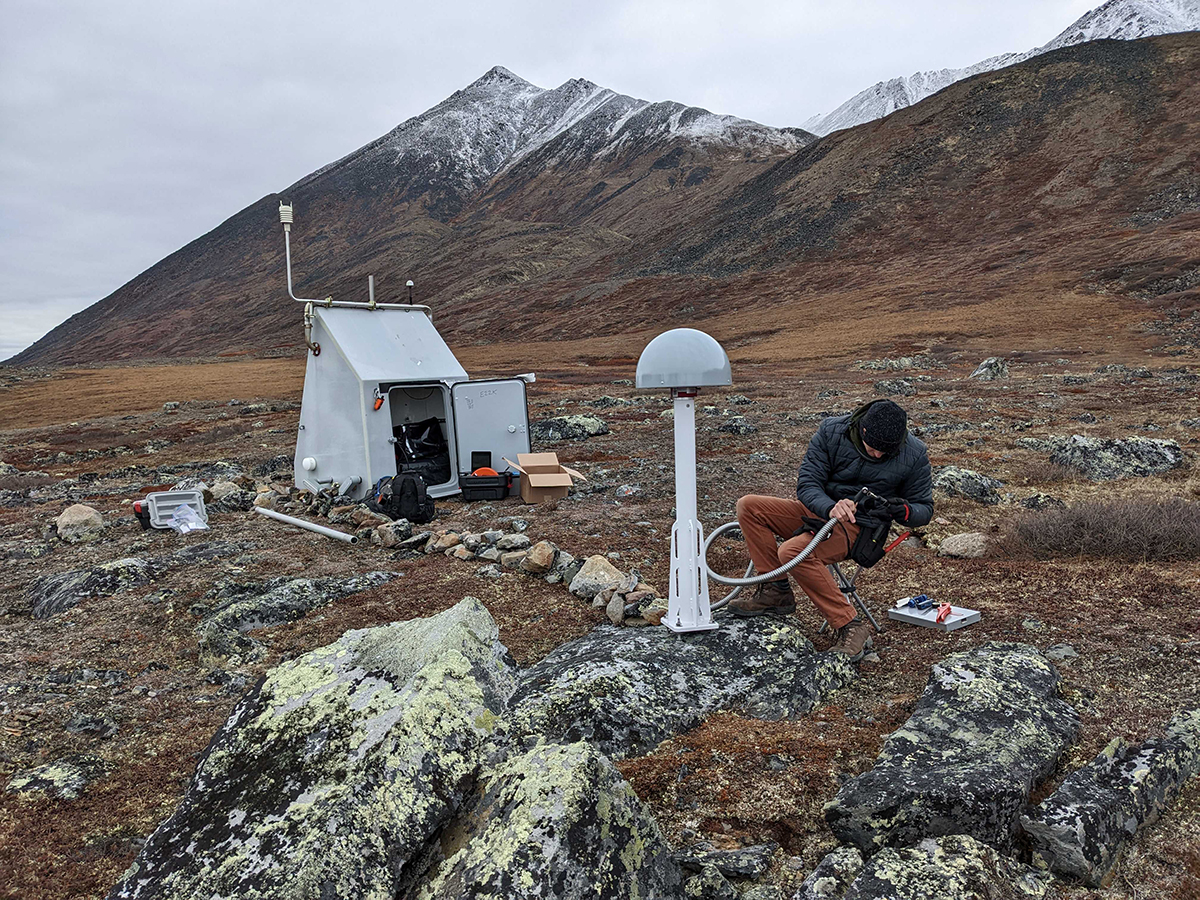
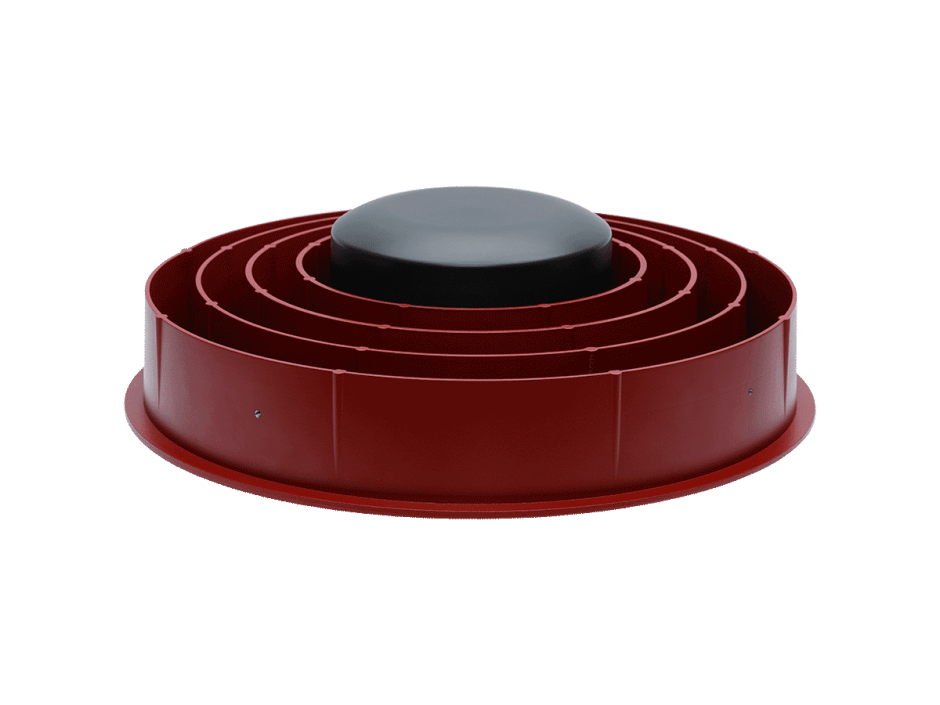
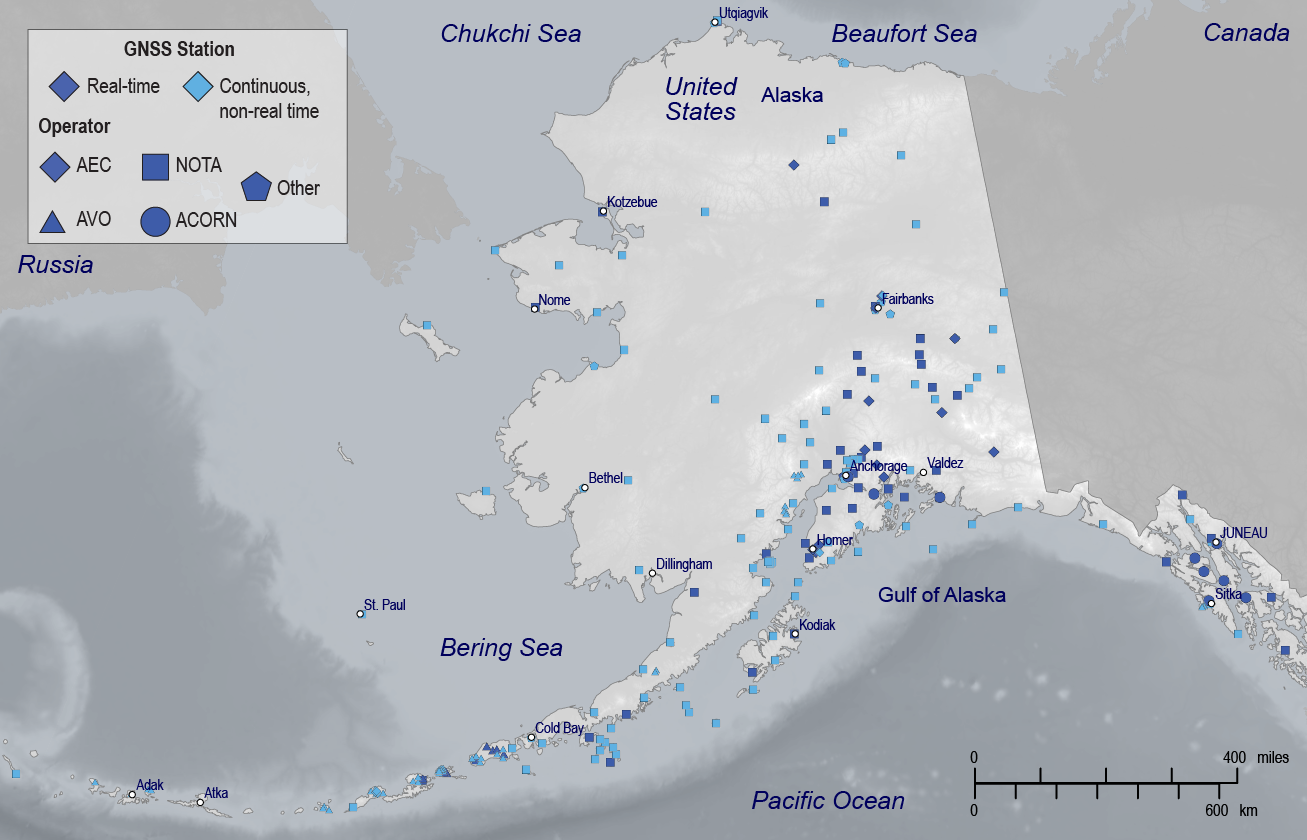
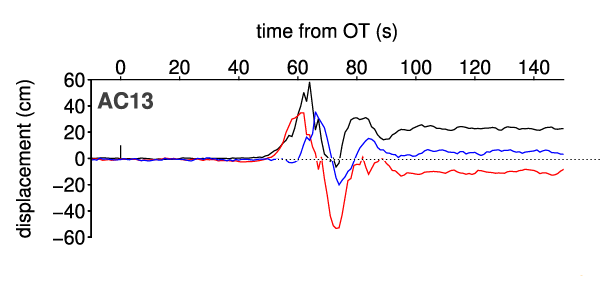
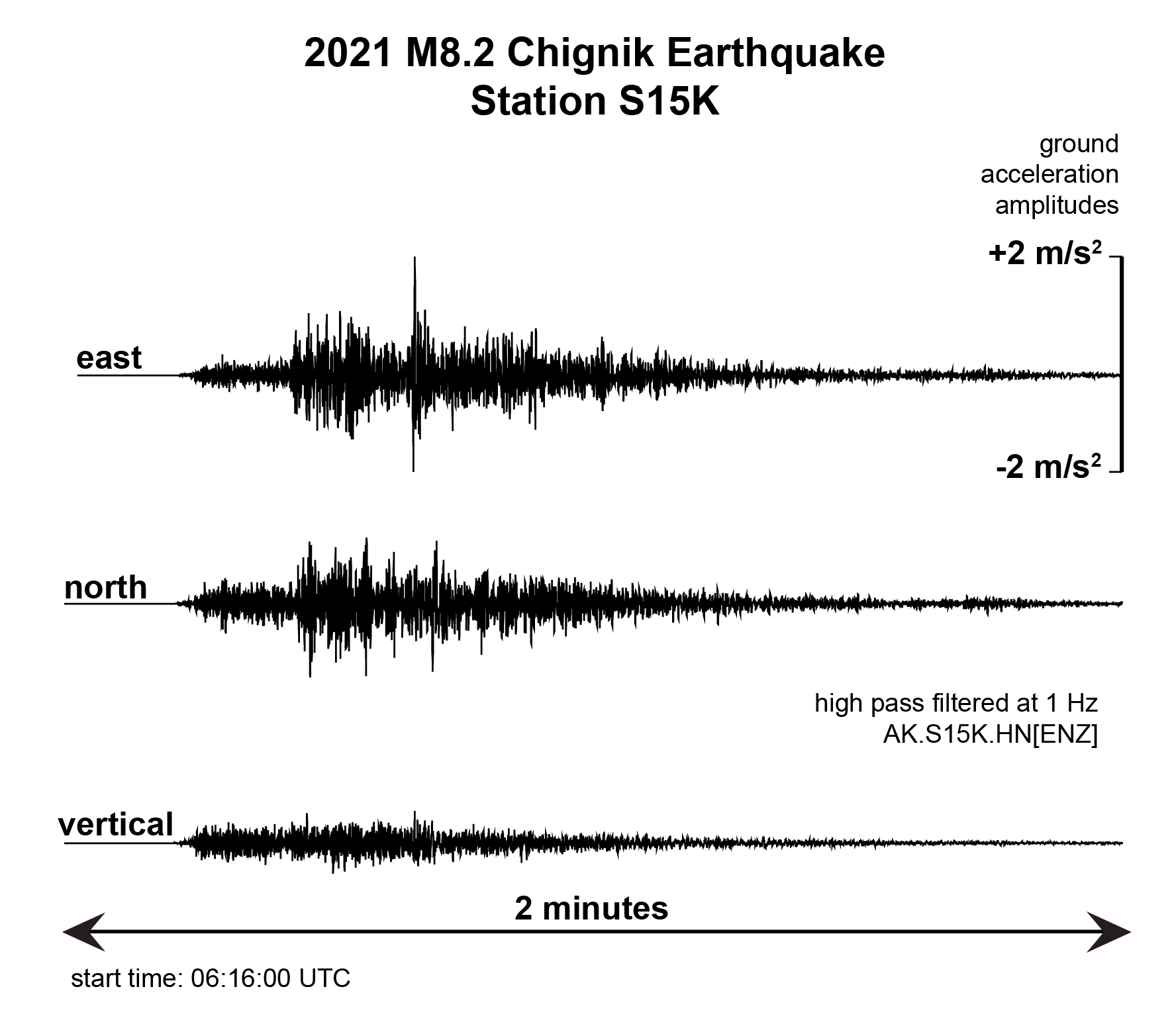
Over the course of several visits to the Alaska Geophysical Network site at Porcupine Dome last summer, Earthquake Center field engineer Cash Koning helped install an instrument that looks like a metal mushroom. It is a Global Navigation Satellite System (GNSS) antenna, which uses satellites to measure shifts in ground position to the scale of millimeters.
GNSS is an expanded GPS system that uses more satellite networks. It offers better accuracy than GPS, the United States satellite constellation, and is increasingly used in seismic monitoring networks.
“Ideally you attach the antenna mount to solid bedrock. The idea is that the mount will move with ground motion,” Koning said. The dome piece is only a protective cover. The actual antenna is made of concentric circles around a small inside dome that protects the computer module. ”It looks like tree rings,” said Koning.
The new addition at Porcupine Dome bumped the number of GNSS instruments the Earthquake Center currently operates up to eight. “We are installing GNSS field equipment, acquiring real-time data here at the Earthquake Center, doing quality control on those data, and archiving and disseminating data to partner organizations,” said Michael West, director of the Earthquake Center.
GNSS and seismic instruments measure different types of information, but they can be used together to generate a better picture of an earthquake. “Seismometers are good at telling us about seismic waves. The GNSS stations are good at telling us short- to long-term movement,” explained Koning. In other words, seismometers measure ground shaking as it happens, but can’t tell you if the ground permanently shifted. A GNSS instrument can measure the distance and direction of ground displacement, which gives us information about the size and movement of an earthquake rupture.
The ground is always moving, sometimes almost imperceptibly slowly, and sometimes suddenly as in an earthquake. GNSS is an important tool for measuring slow-slip ground movements, when faults release strain slowly over weeks or months instead of seconds or minutes like in an earthquake. For example, GNSS networks have measured slow-slip movement in Cook Inlet.
There are a number of GNSS networks in Alaska that measure ground movement: EarthScope’s Network of the Americas (NOTA) is the largest, the Alaska Volcano Observatory has smaller networks near volcanoes, and the Alaska Division of Natural Resources has the Alaska's Continuously Operating Reference Network (ACORN) of real-time stations. NOTA is the backbone of GNSS in Alaska, used for measuring slow tectonic plate motion. It doesn’t transmit data in real time, instead taking measurements about every 30 seconds, then downloading the data once a day. While this is fine for slow-slip events, real-time measurements and data transmission are essential to use GNSS for earthquake early warning or assessing earthquakes as they happen.
The seismometers the Earthquake Center manages transmit data in real time—about as fast as you can text on a cell phone—so we have systems set up to process the data in real time as well. “We had to develop our own system for real-time GNSS data collection and processing,” said Natalia Ruppert, former senior scientist at the Earthquake Center.
GNSS data tends to be “noisier” than that of seismometers. GNSS is best for measuring earthquakes over magnitude 6.0. This is because the earthquake signal (measured as ground displacement) gets masked by the GNSS noise if the amplitude of the earthquake signal is smaller than that of the noise. Earthquakes less than about magnitude 6 do not cause large enough ground displacement for the signal to be distinguished from the GNSS noise. Also, the deeper or farther the earthquake is from the GNSS instrument, the smaller the measurable displacements are on the ground surface.
Despite this, GNSS offers significant advantages. Sensitive broadband seismometers can be overwhelmed by seismic waves from large earthquakes—like blowing out speakers by turning up the volume too loud. This problem is called “saturation”, and results in losing some of the data and making it difficult to determine earthquake magnitude. “GNSS is especially useful for measuring large displacements without saturation. Its accuracy improves with higher sampling rates, which allow it to capture rapid changes in position more precisely. Lower sampling rates may miss some details of fast-moving events,” says Revathy Parameswaran, a UAF Research Assistant Professor who focuses on GNSS and seismology to study earthquakes, volcanoes, crustal deformation, and stress evolution.
Strong-motion seismometers can handle the strongest of shaking. Regardless of the sensor, however, there are fundamental limitations on measuring earthquakes of magnitude 8 or 9 based on the shaking alone near the epicenter. Seismic instruments scattered far away around the globe are capable of distinguishing M9 from M8. However, it can take an hour or more for the seismic waves to reach those stations. This can lead to underestimating the initial magnitude of a large earthquake, which poses significant challenges to emergency activities that happen quickly, such as issuing tsunami warnings.
GNSS measures ground displacement. This means as soon as the earthquake rupture is complete, GNSS has the value for the full displacement caused by the earthquake. The result is that GNSS can estimate the magnitude in tens of seconds to a couple of minutes–about the amount of time that it takes for the fault to rupture. This makes GNSS faster than seismometers at providing a relatively more accurate rapid estimate of magnitude for large earthquakes.
Parameswaran led publication of a scientific paper in the journal Seismological Research Letters, co-authored with Earthquake Center director Michael West, UAF Geodesist Ronni Grapenthin, and PhD student Alex Fozkos. “The main objective with the Chignik paper* was to see how GNSS compares to seismic instruments during a large earthquake—could one be interchanged for the other, or can both be used in tandem?” says Parameswaran. So is Parameswaran Team GNSS or Team Seismometer? “I wouldn’t say one over the other. Both strong-motion and GNSS in any given area would be ideal for quickly assessing earthquakes.”
Newer generations of high-rate GNSS instruments offer faster data collection, making it possible to use GNSS for measuring earthquakes in real time. “A strong-motion seismometer collects 50-200 data samples per second. High-rate GNSS can measure from 1 to 10 data points per second, or even higher frequencies these days, with evolving infrastructure,” says Parameswaran. “That range for GNSS can capture most of the information from large earthquakes used for earthquake early warning.”
Once an earthquake starts, earthquake early warning systems—in place in several countries and U.S. states—warn people before damaging earthquake waves reach them. Rather than predicting, the system relies on a seismic network to (1) detect early, non-destructive seismic waves, (2) rapidly estimate an earthquake location and magnitude, and (3) issue a widespread alert before strong shaking reaches areas away from the epicenter.
As we examine how to implement earthquake early warning in Alaska seismic and GNSS data team up to measure large earthquakes. GNSS instruments complement seismometers for rapid earthquake assessment. While seismometers accurately record shaking, GNSS is superior at measuring substantial ground offsets (sometimes several tens of feet) that occur during the largest earthquakes. GNSS instruments are excellent for measuring the extent of an earthquake rupture. When the ocean floor moves vertically by a significant amount in a coastal region—such as along southern Alaska’s subduction zone—the potential for generating a tsunami is high. A GNSS network can help reduce unnecessary evacuations by more accurately capturing plate motion during the earthquake. Quick assessment by GNSS of significant ground motions will be important in developing our earthquake early warning system.
*This paper may be behind a copyrighted materials paywall. Contact author Revathy Parameswaran at rmparameswaran@alaska.edu if you are unable to access the paper.